by Diane Xue | Apr 13, 2023 | Interviews
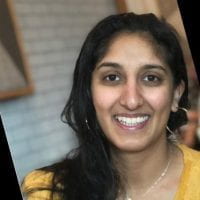
Alyna Khan is a PhD Candidate at the Institute for Public Health Genetics. In addition to being a student, she is also a research scientist at the UW Genetic Analysis Center. Alyna is lead author on “Recommendations on the use and reporting of race, ethnicity, and ancestry in genetic research: Experiences from the NHLBI TOPMed program” published in Cell Genomics this past year. We have invited her to hear about her experiences as a a student and scientist and where she sees her place in the interdisciplinary field of public health genetics.
Diane: IPHG is a unique program, spanning various fields. This program attracts trainees who have interests across genetic epidemiology and the Ethical, Legal, and Social implications of genetics in practice. What were the steps that led you to this interdisciplinary program?
Alyna: I’ve always kind of been interested in so many different topics and subjects. In college, I studied Classics and Poetry and Evolutionary Biology. I feel like I’ve always been pulled to being involved in multiple different fields and learn different perspectives and how they influence each other. So when I learned about this program, it seemed to be such a great fit for me. I didn’t actually take genetics in college, but I learned a lot about genetics through my evolutionary biology classes. Especially in my comparative biology class, I got to learn about different types of organisms, how they’re unique, but also how they’re connected through genetics. I saw classics the same way. It helped me learn about societies’ ideals and institutions, systems, and philosophies.
When I think of public health, I think of so many broad things — not just epidemiology, disease and community health, but also understanding systems: System and policies, systems of justice and law, systems of language, and culture and so on. Public health to me is this big umbrella field where I’m allowed to kind of throw all these things that I’m really fascinated by into one field and also allows me to explore genetics.
After college, I didn’t have a straightforward path, but exposure to genetics was one of the several threads that ran through my work experiences. I started off working at an autism clinic, then moved to a start-up toy company. Directly before coming to UW, I worked at a company that sequenced cancer tumors, analyzing genetic sequences of somatic tumors. And now I’m at the UW Genetic Analysis Center!
Diane: How would you articulate your main research interest now and long-term goals?
Alyna: My main research interests now are around the use of race, ethnicity, and ancestry in genome-wide association studies and polygenic risk scores. The main interest I have is really to get a better understanding of how and why – what the motivations are for using race, ethnicity, and ancestry variables in genetic studies. I’m interested in this because there’s a lot of meaning that can come out of the practice of incorporating those variables into genetics, whether that’s meaning assigned to how those variables are defined, the meaning assigned to the results and interpretation, and how these interpretations are taken up in public understanding.
Diane: Are there particular experiences or courses you’ve had in the program that have shaped your interests or shaped your view of what public health genetics is and where you fit in that interdisciplinary space?
Alyna: Yeah! One of the courses that I’m realizing had a pretty big impact on me was the Genetics and the Law course. I’ve always been really interested in law and in policy making, so I was really excited at the opportunity to get to take a law class, and it was really reassuring to me to see how big of a space genetics can take up in the law. It not only not only made me realize, like, ‘Oh! I have this genetics training, but I can actually work in a more legal, social space.’ I’m realizing I can work in any space with this training, and that’s really cool. I don’t have to be a geneticist who works in a lab at a University. I can apply my training anywhere, including like entertainment and television and policy. Another reason that this class was impactful for me was that Anna (Anna Mastroianni) introduced us to the Innocence Project in that class. I’d heard about it before a little bit, but we had one class where she invited somebody to come in and speak about it. That stuck with me for a while, because learning about the Innocence Project in my Ph. D. program was another specific moment where I thought ‘Oh, I’m capable of working in this space! And the Innocence Project continues to be on my radar for what I want to do after the program.
Similarly pharmacogenetics exposed me to ways in which, having this genetic knowledge, again can open doors for me to work in all sorts of fields that I previously had not thought to be connected to prior to this program.
Diane: Along those lines, are there areas where you think public health and genetics could be more integrated to have a bigger impact on improving population health?
Alyna: I would love to see our program get more involved or provide more opportunities to understand the role of genetics and infectious disease. In 2018, I read about the cholera outbreak in Yemen that was during the Civil War there, and I recall seeing news on genetic sequencing use to trace the cholera outbreak. I remember just being struck by how they were trying to leverage genetics to trace the outbreak, and understand the mutations and the changes. And I know a lot of that had has been going on with Covid, too, but I would love to learn more about how that tracing works. I would love to see our program have more dedicated courses or opportunities like lectures or seminars in that area of genetics and public health. Because we’ve been forced to live through a global pandemic, we’ve seen public health genetics grow and be more in the public mindset. Our program could be positioned to be helpful in those efforts with some additional training opportunities.
Because I’m very interested in law and policy, I do think that there is a lot of potential and great urgency for genetics to be more incorporated in those fields, especially as tech is rapidly increasing and improving and genetics information is become rapidly accessibly. This program highlights the need for policy to keep up with that change – whether that’s in insurance or surveillance or data governance. In the future, as more people have genetic data access, the ELSI (ethical, legal, and social implications) components of our program will only become more important.
Diane: Speaking of ELSI, you were recently a lead author of a paper that provided a set of recommendations for how we should or shouldn’t interpret genetic findings that specifically focuses on delineations between genetic ancestry, race, and ethnicity. This paper describes overlaps and differences between these concepts and offers guidance on when it’s appropriate to use different terms and analysis in order to not conflate these concepts. What was the motivation for writing this paper and how did you get involved?
Alyna: I’ll start with how I got involved. I had overheard my colleagues at the Genetic Analysis Center talking about race and genetics, and I popped my head out of the cubicle and I was like, ‘Hey, you know I clearly was eavesdropping, and I would love to learn more’ and they shared with me that they had started a discussion group at the Genetic Analysis Center to set aside a meeting time where people could discuss topics pertaining to race and genetics. That meeting time was when people would bring up books or articles or podcasts to discuss anything from statistical methods and analytical approaches to working with diverse populations, talking about ethnographies or sociology. There were very rich discussions that touched upon so many different aspects of genetic research from statistical genetics all the way to communication and reporting of genetic studies. I started attending those meetings, and I got involved in the project by volunteering to help draft a set of recommendations. Back at that time we thought ‘Oh, these could just be guidelines for analysts.’ And since at the GAC, we were the data coordinating center for NHLBI’s TOPMed Consortium, we thought it might be helpful to have some guidelines created as a resource for analysts who might be thinking about some of the same questions we were thinking about. So it really just started off as kind of like an organic internal brainstorm as people who have experience working with diverse TOPMed data and being part of the coordinating center. At that time I had been working as part of the phenotype harmonization team. I was gaining experience working with phenotype data, including how we were categorizing and harmonizing race and ethnicity. We were confronted with a lot of challenges in that.
At the same time there were articles and events around that time that were perpetuating ideas of white supremacy and those white supremacist groups were using genetics as justification for their actions and their thoughts and beliefs. That was the year that the American Society of Human Genetics put out a statement denouncing the misappropriation of genetics for white supremacists and so these conversations surrounding race and genetics were happening on a national and global scale, and these recommendations formed as we dug deeper into understanding different perspectives and thinking about how they affected our work.
Diane: You mentioned the possible misappropriation of genetics by white supremacist and others who aim to discriminate on the basis of genetics. I’m guessing these people are probably not going to be the ones looking up this paper and being exposed to these recommendations. In terms of next steps, do you have thoughts about what needs to be done to make these ideas more widely disseminated or reach audiences that aren’t necessarily thinking about how to improve the fields of genetic epidemiology or statistical genetics?
Alyna: I think that’s there’s so many pieces to it. Starting with the genetics community, I think there’s pretty widespread consensus that genetics does not inform race, and vice versa. But I think there’s still a lot of opportunity to iron out the details and the rationale and learn how best to communicate that idea. I think it’ll be important to invite a lot of non-geneticists into that conversation and to be open to understanding what historians and sociologists and anthropologists, et cetera, are saying about race, and what their conceptions of race and biology and ethnicity and ancestry are. And while I’m framing this as like “we as a genetics community invite people in,” I think that exchange, even though I was portraying it as something unidirectional, I think that exchange is bi-directional. Through engaging in that kind of invitation and that kind of conversation with different fields, these ideas will flow between these fields.
There are so many ways in which I try not to talk about genetic exceptionalism. However, I think there are aspects of the genetics field that carry a different burden than other scientific fields in terms of its relationship to (genetics and) race. It’ll be really important for the genetics community to take on and act on the responsibility of decoupling that association. In order to do that, we, as genetic students and scientists and researchers, have to understand and grow professionally with that mindset and communicate with geneticists and non-scientists.
Another thing is to place importance and give credit to is small acts, right. Like for example, not using the word Caucasian in research is a small act that has a big impact. We have a big impact on the language society uses to talk about science and identity. I’m learning about little tweaks one can make in the way that we represent data, like the standards that are set for submitting data tables and their data sets. Even those small acts like ‘Oh, I can’t just say white here. Oh, I need to say country of recruitment, country of birth.’ These are small things. And humans are so resilient; we can get used to new systems.
I just also want to say I feel very impacted by the people around me — whether it’s our PIRS (Progress in Research Seminar) program and Journal Club and PHG students as a whole. My gosh! I don’t know if I can curse on this thing, but like oh, my gosh! Like we know and discuss all these ideas. Opening up more spaces where we can have these conversations will be important. And I just feel so fortunate to be working at the GAC where the people I work with have shared a lot of these ideas or exposed me to the ways that things can be changes. So I’m very grateful for that, too.
Diane : I’m really glad to hear that. It seems like your work environment has provided a pretty inspirational experience.
Alyna: Inspirational and aspirational, yeah. I just feel very lucky to work with them and learn from them.
Diane: It seems like the writing process of this paper was highly collaborative. What was the process of working with a large group of authors?
Alyna: There came to be this desire or potential to eventually turn the guidelines [on reporting of race, ethnicity, and ancestry in genetic research] that we were creating for the consortium into some kind of more formal commentary. While the initial motivation was creating a resource that would be really helpful for TOPMed analysts, there was also a hope that we could turn this into something that would be more broadly accessible. We started with these discussions at the GAC and then reached out to other TOPMed investigators including the executive committee and various committees. We invited anyone who was interested, which helped us build out some specific examples directly from PIs of diverse studies. Then we got an invitation to present the guidelines at ASHG 2020 (American Society for Human Genetics Annual Meeting). We presented it as a talk and got really wonderful feedback from people, one of whom was the editor-in-chief of Cell Genomics, so that sort of started the formal writing process after we got invited to write it as a perspective piece in Cell Genomics. At this point, we reached out to various investigators again. And we really wanted to emphasize that this would represent the views of the authors, not of TOPMed or NHLBI specifically.
I volunteered to do a literature review for the guidelines and took on the role of following up with people who volunteered, organizing logistics and those kinds of things. Then Sarah Nelson and Stephanie Gogarten and Matt Conomos had a lot of the driving ideas, along with other members at the GAC. But because I submitted the abstract to ASHG, when it was accepted as a talk, I was the one to present, and from there, it kind of just blossomed into me being a co-lead (with Stephanie Gogarten) and corresponding author and Sarah took on the role of senior author. In the writing process, my roles included writing, helping with the conceptualization, organization, helping with content, and editing. My area of focus was the harmonization section since that was what I had experience with at work. Logistically, it was also my role to work on setting deadlines and goals, making sure we were hitting those targets, reaching out to co-authors. But we all really did it together- Stephanie, Matt (Conomos), Sarah, and Caitlin McHugh, who’s no longer at GAC. In addition to the input and feedback from others, Sarah, Matt, Stephanie and I split the core writing, editing, conceptualizing- all those different stages, which was really great especially since that was my first time ever participating in all of those roles. I feel really lucky to have that support through this whole process. They always encouraged me any time I had doubts and imposter syndrome about presenting, or being lead author. Getting a PhD can be a very isolating process, but then I got to participate in this very collaborative efforts that felt like a supportive community. It was super cool.
Diane: That is super cool! And I think it’s also great for our students to hear that these collaborative and supportive spaces exist within our academic space. Along that line, what is your number one survival tip that you would give someone starting out in our program? Now, as a fifth-year PhD candidate, what do you wish you had known?
Alyna: I have so many, but I’ll try to pull out the common thread. Having a strong community of friends or family, or chosen family, whatever it is – have a strong community of social support. I that that would have been the number one most helpful thing to have int he PhD. Fortunately I’ve been able to build a little bit of that over time, but again with grad school, it’s so transient. You meet people and they leave, they graduate. So having something that is a constant is something I would have loved to have from the start. The other piece of advice is have one or several forms of escape. Originally for me it was snowboarding in the winter and going to the mountains. Completely disconnect from school and from the city. Lately my major sources of escape and disconnect have been playing Zelda and watching Love is Blind. It is the most ridiculous TV show I’ve ever seen.
Diane: I think these are great tips – it’s really important to remind ourselves and each other that work-life balance is a good thing, especially with balancing student and employee roles.
Alyna: My setup is that I’m an employee at the Genetic Analysis Center, and so I’m not a full time student. I’m not a research assistant, or TA. That has helped me balance. I feel like I’m protected by employee benefits in working twenty hours a week. And so if I want to work more, I can. But if I don’t, I’m protected to work twenty hours a week, and that is like a mental relief. I think knowing that I have that protection as being part of that system of being an employee at the UW — that designation does also remind me that I have a job, and it’s a job that is separate from school. It’s not always an exact divider, especially now in my research days, where I am working with people in my job who are also helping me work on my dissertation. So that line is definitely blurred. But, symbolically, it has helped me maintain a balance between work and school.
Diane: That sounds very healthy. Finally, having lived in Seattle for the past few years, what are three things you recommend? It can be anything.
Alyna:
- The mountains. We live out in this beautiful, beautiful, beautiful place — this is definitely the most beautiful place in the world I’ve ever lived. As students, we have the flexibility to go at off peak times and explore the beautiful nature around us. I’m a mountains person. But obviously out here, if you love water like there’s plenty of that, too.
- Rupee Bar in Ballard. The best cocktails. My favorite place to get cocktails and Sri Lankan snack food. So even if you don’t want to drink anything, they have great vegetarian options and vegan options. A highly recommend!
- Hmmm. Okay. Eating chocolate chip cookies. I’ve had an awesome time applying the skills I’m learning as a PhD student in terms of the depth and planning and logistics of a dissertation to finding my favorite chocolate of cookies in the city. One of the things I’ve been most surprised about living here in Seattle is how many people I’ve met who don’t know what the Metropolitan Market chocolate chip cookie is. It’s a good chocolate chip cookie if you want something rich. And you’re not allergic to nuts. If you want a regular chocolate chip cookie, the chocolate chip cookies at PCC are really good. If you want to up your game, get the dough and bake it yourself. I’ll give you one more. Hello, Robin’s Chocolate chip cookie!
by EJ Dusic and Lex Powers | May 9, 2022 | Genetics and Society
Genetic testing offers an opportunity for individuals to learn more about their risk of developing certain diseases and their genealogy. Both direct to consumer (genetic tests you can order online, such as 23andMe or Ancestry.com) and clinical genetic testing are becoming much more accessible and popular as genetic testing technology advances. As of 2019, more than 2.6 million people had purchased direct to consumer genetic tests in the United States (Regaldo 2019). As genetic technology advances, our understanding of the ethical, legal, and social implications of genetic technology has not kept pace (Phillips et al. 2018; Schaper and Schicktanz 2018), particularly in regard to one process of quality control – the “sex check”.
The sex check is common practice in clinical genetics, direct-to-consumer genetic testing, and genetic research (Mathias et al. 2016; Coleman et al. 2016; Hunter-Zinck et al. 2020; Turner et al. 2011). It involves comparing an individual’s sex chromosomes against their sex or gender. Sometimes, the only information that is available in a clinical or research setting may be either their sex or gender, and it may not be clear which is reported. Although sex and gender are often used interchangeably in genetic research and by genetic scientists, they capture different constructs.
Sex refers to an individual’s sexual chromosome composition or their biological sex characteristics while gender refers to an individual’s internal sense of their gender, whether it be male, female, both, or neither (TSER n.d.). Running the sex check during quality control in genetic testing checks that individuals with a sex or gender that may not be self-reported but is listed as Female have XX chromosomes and individuals with sex or gender that may not be self-reported but is listed as Male have XY chromosomes.
The purpose of this check is to make sure that the sample being processed belongs to the correct individual. Individuals whose sex chromosomes do not match their reported sex or gender are followed up with to ensure the correct sample is being processed for the right person (in the case of clinical or DTC genetic testing) or are removed from a dataset (in a research setting). The utility of this measure is limited; as pointed out by Warmerdam et al. (2021), it does nothing to differentiate sample mix ups between two individuals with the same sex chromosomes. It is only applicable to sample of individuals which contains multiple sexes, and for a sample which contains half people with XX chromosomes and half people with XY chromosomes, it is only 50% effective (Warmerdam et al. 2021). Standard, primary sample quality control procedures in genetic testing include identifying individuals with poor DNA quality, with high variation across autosomal chromosomes, mismatched sex information, removing duplicated or related samples, and running analysis stratified by continental ancestry (Mills, Barban, and Tropf 2020). Additionally, clinical genetic testing, and typically research, involves signing an informed consent at the time that the sample for genetic testing is collected.
Ample procedures are already in place to ensure that the correct sample is matched to the right person. The argument to include the sex-check in genetic testing is weak at best and at its worst it has the effect of either singling transgender individuals or excluding transgender people from genetic research altogether.
Excluding transgender people from genetic research or singling them out in clinical practice directly contributes to the stigma and discrimination that transgender folks experience within the American healthcare system (Institute of Medicine (US) Committee on Lesbian, Gay, Bisexual, and Transgender Health Issues and Research Gaps and Opportunities 2011). Multiple studies have found that transgender individuals do not seek out healthcare services due to previous negative experiences and medical distrust, resulting in decreased healthcare access and worse health outcomes within the transgender community (Barnes, Morris, and Austin 2020; Kosenko et al. 2013; Rolle et al. 2021).
The sex check inherently “others” transgender individuals not only by reminding them of something they may already feel insecure about , but also by perpetuating the idea that biological sex determines gender, a concept tied to genetic determinism (McKeague and Terry 2019). Genetic determinism refers to the belief that our genetic makeup is the sole factor in determining our individual characteristics (Sarraf and Woodley of Menie, Michael A. 2019). This concept has historically been weaponized against the transgender community, particularly in public policy.
An example of the implications of genetic determinism for transgender people and how this ideology results in exclusion of trans individuals lies in the growing national conversation on transgender athletes and whether they should be allowed to participate in competitive sports. Controversial “sex verification,” including molecular tests to identify sex chromosomes, were introduced to determine eligibility for athletes to compete in gendered sporting events in the 1960s (Tucker and Collins 2010). Today, transgender athletes are permitted to compete in the category that is consistent with their gender identity given that they follow certain protocols, such as hormone level monitoring. However, there is still debate as to whether a performance advantage exists for trans women, leading some to argue for reintroduction of genetic testing in this arena. Lia Thomas is the most recent trans athlete to be under public scrutiny for competing in women’s sports (Ghorayshi 2022). This is a reiteration of the same genetic determinism that trans athletes and trans people more broadly have faced for years. Cisgender people see trans people as a threat in sports because of their perceived, unfair advantage due to their biological makeup.
Efforts are being made to move away from genetic determinism (particularly in the clinical space) to be more inclusive of transgender patients, but they often do not go far enough to protect trans people who are receiving clinical care. For example, genetic counselors are currently adapting their practices to be more welcoming and inclusive of folks with varying gender identities. Some of these efforts include use of intake forms that ask for pronouns and gender identity, creation of new pedigree nomenclature that affirms trans and non-binary individuals’ gender identities, and implementation of gender-inclusive language when describing genetics concepts (Barnes, Morris, and Austin 2020; Rolle et al. 2021; Sheehan et al. 2020). Still, an abundance of research describes the negative experiences of trans patients undergoing genetic counseling or genetic testing in a clinical setting. Experiences in a healthcare setting induce anticipatory anxiety for trans people, and it is the responsibility of clinicians to create safety and clarity (Barnes, Morris, and Austin 2020; Rolle et al. 2021).
Clinicians need to clearly communicate with trans patients how and when information about their sex and gender will be used in genetic testing (Barnes, Morris, and Austin 2020). Additionally, it is necessary to take additional steps that validate individuals’ gender identities and to use inclusive language throughout the process of genetic counseling and genetic testing (Barnes, Morris, and Austin 2020; Rolle et al. 2021).
Genetic testing companies are taking steps to be more inclusive by creating “best practices” for processing genetic tests for their transgender clients. For example, Color Genomics has integrated questions about gender identity and sex assigned at birth into their health history intake, as well as a disclaimer that gendered information within a genetic test report may not reflect their identity (Mar et al. 2020). Direct-to-consumer genetic testing company 23andMe allows for customers to self-report their “profile sex” with which they identify, and advise that during sample processing if “genetic sex” does not match selected profile sex they will notify the customer, who can validate that the difference was expected (23andMe n.d.). Other genetic testing companies also notify or call patients when this occurs to ask them to confirm their sex assigned at birth. This process of validating the difference between profile sex and chromosomal sex, in practice, singles out trans people by creating an incredibly uncomfortable experience and presenting an additional barrier to care.
Another concern in the process of genetic testing lies in the return of results. Genetic testing reports that make suggestions for preventative care are inherently gendered. For example, a positive result for a BRCA1 mutation may state that women with a mutation should receive regular mammograms without considering that not all individuals with breasts may identify as women. For both 23andMe and Color Genomics, the test report that is returned to consumers still contains gendered information, such as labels like “maternal” or “paternal”, no matter how the customer or their relatives identify. Altogether, the consequence of the practices of these genetic testing companies are only somewhat successful in preventing negative experiences or interactions with their transgender clients.
To make genetic testing more approachable and more inclusive to individuals whose gender identities don’t match their sex chromosomes, we need to carefully consider how the sex check is being used and exactly how necessary it is. Given all of this information, we make the following recommendations:
First, we call for genetic testing companies and genetic researchers to think carefully about the necessity of the sex-check, and to implement alternative quality control procedures when appropriate. While alternative methods compare other phenotypic characteristics with a sample’s genotype, new methods which rely on the generation of polygenic risk scores have been shown to be more effective in identifying sample mix ups (Warmerdam et al. 2021).
When necessary, researchers and genetic testing companies should utilize and collect information on both sex assigned at birth and gender identity to ensure that individuals whose chromosomes do not match their reported sex are not singled out in the genetic testing process.
Additionally, we suggest updating language in the genetic testing process to be more inclusive, such as changes to pedigree nomenclature and avoidance of gendered language such as “maternal” or “paternal”. Moving forward, it is our responsibility as genetics professionals to address the historical exclusion and discrimination of trans people within the fields of clinical and research genetics and to ensure that an individual’s genetic makeup will not be weaponized against them.
References
Barnes, Heather, Emily Morris, and Jehannine Austin. 2020. “Trans-Inclusive Genetic Counseling Services: Recommendations from Members of the Transgender and Non-Binary Community.” Journal of Genetic Counseling 29 (3): 423–34.
Coleman, Jonathan R. I., Jack Euesden, Hamel Patel, Amos A. Folarin, Stephen Newhouse, and Gerome Breen. 2016. “Quality Control, Imputation and Analysis of Genome-Wide Genotyping Data from the Illumina HumanCoreExome Microarray.” Briefings in Functional Genomics 15 (4): 298–304.
De Sloover D. Servais L. Zhou A. & Topper S., Mar L. Rieger K. Helm Stamp M. Musselman-Brown A. . 2020. “Designing for Inclusivity in Population Genomics.” Color Genetics. 2020. https://www.color.com/wp-content/uploads/2020/05/2020_ACMG_Poster_Gender_final.pdf.
Ghorayshi, A. 2022. “Trans Swimmer Revives an Old Debate in Elite Sports: What Defines a Woman?” The New York Times, 2022.
Hunter-Zinck, Haley, Yunling Shi, Man Li, Bryan R. Gorman, Sun-Gou Ji, Ning Sun, Teresa Webster, et al. 2020. “Genotyping Array Design and Data Quality Control in the Million Veteran Program.” American Journal of Human Genetics 106 (4): 535–48.
“I’m Transgender. Will This Impact My DNA Test Kit Experience?” n.d. 23andMe. Accessed February 20, 2022. https://customercare.23andme.com/hc/en-us/articles/115015843628-I-m-Transgender-Will-This-Impact-My-DNA-Test-Kit-Experience-.
Institute of Medicine (US) Committee on Lesbian, Gay, Bisexual, and Transgender Health Issues and Research Gaps and Opportunities. 2011. The Health of Lesbian, Gay, Bisexual, and Transgender People: Building a Foundation for Better Understanding. Washington (DC): National Academies Press (US).
Kosenko, Kami, Lance Rintamaki, Stephanie Raney, and Kathleen Maness. 2013. “Transgender Patient Perceptions of Stigma in Health Care Contexts.” Medical Care 51 (9): 819–22.
Mathias, Patrick C., Emily H. Turner, Sheena M. Scroggins, Stephen J. Salipante, Noah G. Hoffman, Colin C. Pritchard, and Brian H. Shirts. 2016. “Applying Ancestry and Sex Computation as a Quality Control Tool in Targeted Next-Generation Sequencing.” American Journal of Clinical Pathology 145 (3): 308–15.
McKeague, Madelyn, and Sharon F. Terry. 2019. “Let’s Talk about Sex: Understanding Gender beyond Genetics.” Genetic Testing and Molecular Biomarkers 23 (9): 599–600.
Mills, Melinda C., Nicola Barban, and Felix C. Tropf. 2020. An Introduction to Statistical Genetic Data Analysis an Introduction to Statistical Genetic Data Analysis. The MIT Press. London, England: MIT Press.
Phillips, Kathryn A., Patricia A. Deverka, Gillian W. Hooker, and Michael P. Douglas. 2018. “Genetic Test Availability and Spending: Where Are We Now? Where Are We Going?” Health Affairs (Project Hope) 37 (5): 710–16.
Regaldo, A. 2019. “More than 26 Million People Have Taken an At-Home Ancestry Test.” MIT Technology Review, 2019.
Rolle, Lathel, Kimberly Zayhowski, Diane Koeller, Dee Chiluiza, and Nikkola Carmichael. 2021. “Transgender Patients’ Perspectives on Their Cancer Genetic Counseling Experiences.” Journal of Genetic Counseling, December. https://doi.org/10.1002/jgc4.1544.
Sarraf, Matthew A., and Woodley of Menie, Michael A. 2019. “Genetic Determinism.” In Encyclopedia of Evolutionary Psychological Science, edited by Todd K. Shackelford and Viviana A. Weekes-Shackelford, 1–14. Cham: Springer International Publishing.
Schaper, Manuel, and Silke Schicktanz. 2018. “Medicine, Market and Communication: Ethical Considerations in Regard to Persuasive Communication in Direct-to-Consumer Genetic Testing Services.” BMC Medical Ethics 19 (1). https://doi.org/10.1186/s12910-018-0292-3.
Sheehan, Elizabeth, Robin L. Bennett, Miles Harris, and Gayun Chan-Smutko. 2020. “Assessing Transgender and Gender Non-Conforming Pedigree Nomenclature in Current Genetic Counselors’ Practice: The Case for Geometric Inclusivity.” Journal of Genetic Counseling 29 (6): 1114–25.
Trans Student Educational Resources. n.d. “The Gender Unicorn.” Transforming Education. Accessed April 27, 2022. https://transstudent.org/gender/.
Tucker, Ross, and Malcolm Collins. 2010. “The Science of Sex Verification and Athletic Performance.” International Journal of Sports Physiology and Performance 5 (2): 127–39.
Turner, Stephen, Loren L. Armstrong, Yuki Bradford, Christopher S. Carlson, Dana C. Crawford, Andrew T. Crenshaw, Mariza de Andrade, et al. 2011. “Quality Control Procedures for Genome-Wide Association Studies.” Current Protocols in Human Genetics / Editorial Board, Jonathan L. Haines … [et Al.] Chapter 1 (January): Unit1.19.
Warmerdam, Robert, Pauline Lanting, Lifelines Cohort Study, Patrick Deelen, and Lude Franke. 2021. “Idéfix: Identifying Accidental Sample Mix-Ups in Biobanks Using Polygenic Scores.” Bioinformatics (Oxford, England) 38 (4): 1059–66.
by slewan | Mar 23, 2021 | Genetics and Society
In recent years direct-to-consumer (DTC) genetic tests have become so accessible, becoming something of a cultural phenomenon. As of 2021 there are more than 77,000 different types of genetic tests being performed around the world for more than 10,000 different conditions with more than 300 labs performing these tests in the U.S alone [1]. By 2019, 26 million Americans had taken part in DTC genetic testing, through companies such as FamilyTree DNA, Ancestry.com, and 23andMe [2].
Today, using these tests, I could find out that I carry a mutation in the BRCA1 gene. The presence of this mutation means that I would have more than a five-fold increase in my lifetime risk for breast cancer and more than a thirty-six fold increase in my lifetime risk for ovarian cancer [3]. The good news is that equipped with the knowledge of this mutation, I could take life-saving preventive measures to mitigate that cancer risk [4]. The bad news is that in some states a variety of companies can now use this genetic information to legally discriminate against me if I were to apply for some type of economic benefit, such as a mortgage or life insurance. These companies can request my genetic test result and use the result to deny my application altogether, to justify setting different terms of agreement, or deny my application if I refuse to give them the information.
The only federal law that prohibits discrimination on the basis of one’s genetics prior to the manifestation of a disease or disorder is the 2008 Genetic Information Nondiscrimination Act (GINA) [5]. One of the intentions of GINA is to allow Americans to take advantage of personalized medicine, like testing for the BRCA1 genetic mutation, without fear of discrimination [6]. GINA defines “genetic information” as an individual’s genetic tests, the genetic tests of family members, and the manifestation of disease in family members [5]. GINA provides significant protections against genetic discrimination in employment and health insurance settings. Title I of GINA prohibits health insurers from using genetic information to determine an individual’s eligibility or coverage, underwriting, or premium-setting decisions [5]. Title I also prohibits health insurers from requesting or requiring individuals or their family members to undergo genetic testing or provide genetic information [5]. These protections extend to private insurers, Medicare, Medicaid, Federal Employees Health Benefits, and the Veterans Health Administration [7-8]. Title II of GINA prohibits employers from using genetic information in employment decisions and requiring or requesting genetic information from applicants or employees [5].
While the protections GINA provides are important, they don’t go far enough. It is unlikely that at the time of GINA’s passage in 2008 the government anticipated the DTC genetic testing boom. GINA does not provide protections in a wide variety of important scenarios. For instance, GINA does not ban genetic discrimination in any other realms outside of employment and health insurance. This leaves out different types of insurance coverage, including long term care, disability, and life insurance [9-10], as well as applications for housing, education, or mortgage lending [10]. It is legal in some states for a mortgage lender to request genetic information from an applicant to estimate if they will be healthy long enough to pay off their loan. The mortgage lender can then use this information to decide if they are going to deny a loan application altogether, set higher interest rates, or set shorter loan terms for those with genetic information that suggests they are genetically predisposed to being unhealthy. These companies have the right to deny your application based on your test results or if you don’t provide them with the genetic information they want.
Furthermore, GINA only protects those who have not yet manifested a disease, even though the distinction between an asymptomatic individual and an individual that has manifested a disease is frequently ambiguous [11]. Those who have already manifested a genetic condition must rely on other laws, like the Americans with Disabilities Act (ADA), for legal protection [7]. GINA also does not apply to everyone. GINA does not apply to employers with fewer than 15 employees and only applies to the military in part [5]. GINA protects military members from discrimination in the military’s TRICARE health insurance program. However, one must be a member of the military to enroll in TRICARE and the GINA does not prohibit the military from using genetic information to make employment decisions [8]. However, military policies are in place that mirror GINA in many ways [9]. Interestingly, the Department of Defense (DoD) has officially advised all military members to refrain from direct-to-consumer genetic testing [12], which is counterintuitive to one of the previously mentioned underlying purposes of GINA, to encourage people to take advantage of genetic testing without fear of discrimination [6].
Some states have recognized these shortcomings and have enacted state level legislation to provide additional protections within their borders, but the protections they offer vary widely in scope from state to state [8]. In 2011, California enacted Cal-GINA, one of the most inclusive genetic nondiscrimination state statutes [8,13]. Cal-GINA expanded protections against genetic discrimination in housing, employment, education, public accommodations, health insurance coverage, life insurance coverage, mortgage lending, elections and to employers with 5 or more employees [13]. Cal-GINA amended the Unruh Civil Rights Act to make genetic information a protected class [14] outlawing genetic discrimination in “all business establishments of every kind whatsoever” [15]. Cal-GINA also allows for individuals to seek unlimited damages, which the federal GINA caps at $50,000-$300,000 dependent upon the size of the defendant [16]. In contrast, Maryland passed one of the least inclusive genetic nondiscrimination state statutes in 2008. Maryland House Bill 29 stated that if a long-term care insurance company can provide evidence that the genetic test result would be useful to them financially, they can request and use it to determine insurance eligibility and premiums [17]. Moreover, this bill did not provide protections for either life or disability insurance.
According to a 2012 analysis, 13 states had restricted the use of genetic information in life insurance, 14 states had restricted it’s use in disability insurance, and 11 states had restricted it’s use in long-term care insurance [18]. Only a handful of states had laws that regulated all three types of insurance [18]. Ultimately, this patchwork of legislation is confusing and contributes to American’s fears and concerns surrounding genetic testing and discrimination. The interstate variability in genetic nondiscrimination laws makes it difficult for consumers of genetic testing to navigate and are insufficient to deal with the widespread use of genetic testing, including both clinical and DTC genetic testing, across all 50 states.
GINA needs to be expanded at the federal level to provide consistent nationwide protections to Americans. With so many ways in which genetic information can be misused, federal genetic nondiscrimination legislation must be broad [14]. Therefore, GINA should be expanded at the federal level to mimic Cal-GINA by extending genetic discrimination protections to housing, employment, education, public accommodations, health insurance coverage, life insurance coverage, mortgage lending, elections, employers with 5 or more employees [12] and in “all business establishments of every kind whatsoever” [15]. This would truly provide comprehensive protection from genetic discrimination to all Americans.
The longer GINA remains unchanged more Americans will face genetic discrimination. This may contribute to Americans forgoing genetic testing, both DTC and medically recommended, out of fear that they will later face genetic discrimination as a result. Additionally, Americans may forgo participating in genetics research for the same fearful reasons, ironically leading to stagnation in this field of science, and ultimately slowing progress towards the cures and treatments of the genetic diseases we are discriminating against.
My ask is simple: do some more research yourself and start informing your friends, family, and colleagues about this issue. Then, request that your representatives get familiar with this issue so they can advocate for the expansion of GINA at the federal level to mimic Cal-GINA to widely prohibit genetic discrimination throughout the U.S. Here are some resources to help you get started.
References
[1] Rubinstein, W. S., Maglott, D. R., Lee, J. M., Kattman, B. L., Malheiro, A. J., Ovetsky, M., … Ostell, J. M. (2012). The NIH genetic testing registry: a new, centralized database of genetic tests to enable access to comprehensive information and improve transparency. Nucleic Acids Research, 41(D1), D925–D935. https://doi.org/10.1093/nar/gks1173
[2] Regalado, A. (2020, June 19). More than 26 million people have taken an at-home ancestry test. Retrieved December 13, 2020, from https://www.technologyreview.com/2019/02/11/103446/more-than-26-million-people-have-taken-an-at-home-ancestry-test/
[3] Kuchenbaecker, K. B., Hopper, J. L., Barnes, D. R., Phillips, K.-A., Mooij, T. M., Roos-Blom, M.-J., … Olsson, H. (2017). Risks of Breast, Ovarian, and Contralateral Breast Cancer for BRCA1 and BRCA2 Mutation Carriers. JAMA, 317(23), 2402. https://doi.org/10.1001/jama.2017.7112
[4] Kotsopoulos, J. (2018). BRCA Mutations and Breast Cancer Prevention. Cancers, 10(12), 524. https://doi.org/10.3390/cancers10120524
[5] Genetic Information Nondiscrimination Act of 2008. (n.d.). Retrieved November 21, 2020, from https://www.congress.gov/bill/110th-congress/house-bill/493/text
[6] Suter, S. M. (2018). GINA at 10 years: the battle over ‘genetic information’ continues in court. Journal of Law and the Biosciences, 5(3), 495–526. https://doi.org/10.1093/jlb/lsz002
[7] Congressional Research Service. (2015, August). The Genetic Information Nondiscrimination Act of 2008 (GINA)(RL34584). Retrieved from https://crsreports.congress.gov/product/pdf/RL/RL34584
[8] National Human Genome Research Institute. (2020a, August 3). Genome Statute and Legislation Database. Retrieved November 20, 2020, from https://www.genome.gov/about-genomics/policy-issues/Genome-Statute-Legislation-Database
[9] Baruch, S., & Hudson, K. (2008). Civilian and Military Genetics: Nondiscrimination Policy in a Post-GINA World. The American Journal of Human Genetics, 83(4), 435–444. https://doi.org/10.1016/j.ajhg.2008.09.003
[10] Zhang, S. (2017, March 13). The Loopholes in GINA, the Law Prohibiting Genetic Discrimination. The Atlantic. Retrieved from https://www.theatlantic.com
[11] Areheart, B. A., & Roberts, J. L. (2019). GINA, Big Data, and the Future of Employee Privacy. Yale Law Journal, 128(3), 710–790. Retrieved from https://advance-lexis-com.offcampus.lib.washington.edu/api/document?collection=analytical-materials&id=urn:contentItem:5VCK-7150-02BN-10RB-00000-00&context=1516831.
[12] Department of Defense. (2019, December). Direct-to-Consumer Genetic Testing Advisory for Military Members. Retrieved from https://www.scribd.com/document/440727436/DOD-memo-on-DNA-testing#from_embed
[13] Senate Bill No. 559, Chapter 261. (2011, September 6). Retrieved November 21, 2020 from https://leginfo.legislature.ca.gov/faces/billNavClient.xhtml?bill_id=201120120SB559
[14] Prince, A. E. R. (2013). Comprehensive Protection of Genetic Information : One Size Privacy or Property Models May Not Fit All. Brooklyn Law Review, 79, 175. Retrieved from https://advance-lexis-com.offcampus.lib.washington.edu/api/document?collection=analytical-materials&id=urn:contentItem:5BSP-GBB0-00CV-M0N8-00000-00&context=1516831.
[15] Unruh Civil Rights Act. (1959). Retrieved from https://leginfo.legislature.ca.gov/faces/codes_displaySection.xhtml?lawCode=CIV§ionNum=51
[16] Rothstein, M. A., & Rothstein, L. (2017). FEATURE, THE USE OF GENETIC INFORMATION IN REAL PROPERTY TRANSACTIONS, 31 Probate & Property 13. Retrieved January 10, 2021, from https://advance-lexis-com.offcampus.lib.washington.edu/document?crid=39b943d7-95ff-4fd4-9b62-f69306140616&pddocfullpath=%2Fshared%2Fdocument%2Fanalytical-materials%2Furn%3AcontentItem%3A5P09-Y730-00DB-537M-00000-00&pdsourcegroupingtype=&pdcontentcomponentid=155878&pdmfid=1516831&pdisurlapi=true#
[17] House Bill 29. (2008, May 22). Retrieved November 23, 2020, from http://mgaleg.maryland.gov/mgawebsite/search/legislation?target=/2008rs/billfile/hb0029.htm
[18] Beyond GINA, States Build Patchwork of Protections. (2012, August 22). Retrieved November 23, 2020, from https://www.genomeweb.com/archive/beyond-gina-states-build-patchwork-protections#.X7w0vy9h0_V
[19] National Human Genome Research Institute. (2020b, September 16). Genetic Discrimination. Retrieved November 22, 2020, from https://www.genome.gov/about-genomics/policy-issues/Genetic-Discrimination
by Yunqi Li | Jan 13, 2021 | Interviews
Dr. Taryn Hall is an alumna of the Institute of Public Health Genetics Ph.D. program. After graduating in 2016, she trained as a senior fellow and then a research scientist at the Department of Biomedical Informatics and Medical Education at UW for three years. In 2019, she started her position as a Principal Research Scientist in Genomics at the UnitedHealth Group in Minnesota. We invited Taryn to a virtual interview and asked about her experience in the industry, perspectives of the public health genetics field, and advice for current and prospective students.
Q: Where do you currently work?
A: I work at what’s now called OptumLabs, which is the research and development arm of UnitedHealth Group. It is a little bit interesting to talk about: United Health Group is a really large healthcare organization, and it breaks into a couple of different parts. Underneath this parent organization, there is United Healthcare, an insurer, and Optum, which provides clinical services. At OptumLabs, we are trying to find innovative ways to get better healthcare to as many people as possible for a lower cost.
Q: What are your job responsibilities?
A: I am a Principal Research Scientist. I actually do two things. First, I do a lot of strategy work. I am writing to the business audience. I have to do pieces of writing that express a point of view on some genomic technology and develop good ideas of how we use any new technology or whatever to improve health. For example, a recent interest for our businesspeople is polygenic risk scores. I wrote a piece to help our business team understand what polygenic risk scores are, how they could be used, and their limitations, and I make recommendations. Secondly, I also do some technical work. I am conducting proof-of-concept studies. The projects are usually used to test a key assumption that we have about a business that we want to create.
Q: What are the rewarding and challenging part of your work?
A: The rewarding part is that I can get to be as creative as I want to be. I have a lot of resources at my disposal. My senior leadership really rewards just thinking outside the box. If you can envision a future that you want to be, you have pretty well carte blanche to work towards that. OptumLabs started as a tiny start-up, and they have a very maverick sort of culture. There is not a lot of process and procedure as they grow bigger. So, I think the most challenging thing is finding the process to get the people you need or get the resources you need or get something done, just because the channels change every three months here. It is a very fast-changing environment, which you have to learn to roll with that.
Q: What is the promotion path in your job?
A: Postdoc -> principal research scientist -> senior principal research scientist -> fellow. As a fellow, you are taking your own little research group, and you have a focus area of your own. It is a flat organization, so there is not a lot of places to move beyond fellow. We have some VPs, a management role, but I do not know that one would necessarily go to that level. One probably would transition somewhere else within UHG or outside the company.
Q: What are some important questions you found in the public health genetics field?
A: I am interested in how to refine penetrance of either rare or common diseases. We lose a lot of precision in our financial models due to reduced penetrance. So, looking for ways of combining other data streams, e.g., polygenic marker, monogenetic marker, or environmental data, or anything else to refine that penetrance value could better get people to the care they need when they need it. And then we are also looking at heritable cancer, e.g., breast cancer, Lynch syndrome. Current interventions are really burdensome, especially for people with heritable cancer risk who are in their childbearing and rearing years. Is there any technology that can be used are not quite cumbersome or invasive? And are there other things that we can for prophylaxis other than cutting off body parts? I am also interested in discovering other biomarkers that can be used to track disease progression. If knowing there is a trend towards developing a certain condition, one could do some lifestyle-wise intervention that could bring back into the normal stage.
Q: Which course/course series in PHG at UW helps you most in your work?
A: Probably the technology-focused ethics course taught by Prof. Fullerton. My job really values being able to have a strong position on the topic. A lot of writing that I am doing is defending you position, like “we should do this”, which you have to be able to back up and have a strong argument behind it. This course, and also even PHG512, covers a broad overview of all of the different pieces that involved in public health genetics. I use that all time to look at a problem from different angles, e.g., “Here are all the things we have to look for” “These are our risk point” “We need to pay attention to these areas”. PHG focuses a lot on what problems could arise, but it also trains you to think about how you can use these to be innovative and how it could benefit people as well.
Q: What resources outside PHG at UW helps you most in your work?
A: I did a postdoc in the bioinformatics program at UW, and that was really another great skillset to pick out for what I do. I am working with a lot of medical data and claims data. Knowing what can be done with this data under the structure is really helpful.
Q: What is the most valuable thing you learned or took away from the PHG program?
A: Being able to tackle a problem from multiple different angles and learning the value of bringing different voices to the table and seeing all these different perspectives.
Q: What was the most challenging part of your graduate studies?
A: I was in a class where things got a little bit hairy politically with the program, so it was actually hard for me to get graduate because there were so many changes going on. There is a big change in the director and my advisor. My advisor was leaving and went to a different university right after I passed my general exam. I basically started over with it all and finished under a different advisor by changing my topic entirely. So, I lost about three years. If I had to do it again, I need to advocate for myself more. I did not know how to work with faculty to get things done. They pulled in so many different directions and were not necessarily thinking about you unless you bring it to their attention. I think my advice would be to be proactive about getting what you need from your advisers.
Q: Given that we are an interdisciplinary program, I sometimes feel like we are trained to “know a little bit of everything”. However, in the job markets, most of the positions are looking for a candidate with solid training in a specific professional ability, e.g., statistical genetics, genetic epidemiology, health economics, health policy management, etc. How did you develop your professional skills to be competitive in the market?
A: Looking at where you want to be and what you are good at. I knew I was a good writer and teacher. Also, I never shut down or closed any doors. Besides writing, I also have a strong analytic side. There are a lot of data science jobs. Having analytical skills is definitely a good marketplace fallback. Otherwise, communication and project managing skills can serve you in both industries and academia.
Q: Any other suggestions you would share with a current or prospective student?
A: Be more proactive with getting what you needed. Not falling into the trap of having imposter syndrome. Having confidence that you are where you are for a reason. Keep working, building your skills, and acquiring new skills.
by Emily Hasser | Dec 22, 2020 | Disease Genetics, Evolution
Main Takeaways:
- Genetic mutations that lead to uncontrolled cell proliferation can cause cancerous tumors. Random genetic mutations occur at every cell division. As more cell divisions accumulate, there are increased chances for a cancer-causing mutation. While one would expect that larger organisms would be at an increased risk for cancer because they accumulate greater amounts of cell divisions, this is not the case for elephants and whales.
- Several hypotheses may explain this paradox: shorter telomeres, negative selection against hypertumors, and variation in the TP53 gene. Read below for more detail.
In the United States, the National Cancer Institute estimates that about 1.8 million new cancer diagnoses and approximately 600,000 cancer-related deaths will occur in 2020 [1]. Furthermore, about 39.5% of people can expect to receive a cancer diagnosis at some point in their life [1]. Although cancer has many possible causes, downstream genetic mutations ultimately drive the development of cancer [2].
In multicellular organisms, genetic mutations don’t just affect a single, isolated cell, but can be passed on to many cells in the organism through cell division as the organism grows. Random genetic mutations occur at every cell division, and some of these can lead to uncontrolled cell proliferation resulting in the growth of tumors that can become cancerous. As an organism grows larger and lives longer, more cell divisions will occur in its body over its lifetime. One might expect that larger organisms would accumulate more mutations due to increased numbers of cell divisions and that there should be more chances for tumor growth. However, mortality due to cancer in large animals with long lifespans is not higher than in humans: this discrepancy is known as Peto’s Paradox [3]. If organisms the size of whales had up to 1,000 times the cancer risk than humans, as we might expect given the increased number of cell divisions and random mutations, it would be very unlikely for them to reproduce before succumbing to cancer.
Several hypotheses have been proposed to account for Peto’s Paradox, using data collected from large animals such as whales and elephants [3,4]. Studying cancer in wild mammals presents unique challenges due the lack of accessibility to populations and inability to control factors such as environmental exposures. However, whales, porpoises, and dolphins are all cetaceans, which is a group of wild animals that has been extensively studied [4]. Except for a few cases linked to environmental pollution, cancer in whales rarely occurs [3,4]. This makes them a great model organism for studying Peto’s Paradox. Since these organisms do not appear to have a high risk of cancer, it is suggested that their cancer prevention mechanisms are likely more effective than those of smaller organisms. Other researchers maintain that the rate of cancer development is the same, but cancer may not be as lethal in larger organisms. Here, I will describe hypotheses explaining Peto’s Paradox involving telomeres, hypertumors, and the tumor suppressor gene p53, in the order of least to most investigated.
Telomeres
Telomeres may play a critical role in cancer suppression in large organisms. Similar to how shoelaces have plastic aglets to prevent fraying, telomeres are repetitive DNA sequences located on both ends of each chromosome to prevent DNA damage [5]. Telomeres protect the important stretches of the genome located in the middle of the chromosomes and have recently become a hot topic in research due to possible implications in aging and cancer [5].

Telomeres (pictured in purple) gradually shorten each time a cell divides.
Telomeres deplete with every round of DNA replication. When the telomeres become too short, the cell becomes senescent, entering a dormant state in which it doesn’t divide. Researchers hypothesize that shortened telomeres in large organisms with long lifespans could explain their reduced cancer incidence [3]. Reducing the number of times an individual cell divides would reduce the opportunities for a mutation in an oncogene or tumor suppressor gene to occur. With fewer previous divisions for mutations to accumulate, the cell has a decreased risk of developing cancer. The exploration of telomere modifications as an explanation for Peto’s Paradox has just begun, and further research is needed to investigate the possibility.
Hypertumors
Another new hypothesis proposed to explain Peto’s Paradox is promising, but has only been illustrated in silico, or through a computer simulation. Researchers suggest that in larger animals, malignant tumors have a fitness disadvantage compared to benign tumors [4]. In a population of cancer cells with various phenotypes, natural selection may favor aggressive “hypertumors” that piggyback off the vascular growth of parent tumors. Acting as parasites, these hypertumors deplete the parent tumor’s resources and eventually destroy it. Unlike in small organisms, tumors need to reach a substantial size to have consequences in large organisms. Therefore, hypertumors have plenty of time to develop and damage the original tumor before the original tumor grows to a lethal size. As a result, cancer may still be more common in large organisms, just less lethal [4]. Additional research investigating tumor growth in living whales is needed before any concrete conclusions can be drawn.
TP53
Variation in TP53 has been identified as another possible explanation for Peto’s Paradox [3,6]. As a tumor suppressor gene, TP53 helps control cell growth, and mutations in the TP53 gene have been found in up to 50% of human cancers. The p53 protein expressed by this gene has primary roles in cell cycle arrest, DNA repair, and apoptosis. Mutations in the TP53 can lead to reduced expression of p53 and the uncontrolled cell growth that is a hallmark of cancer [7].
Imagine the cell cycle as the process of loading laundry into your washing machine. You’ve put the clothes and detergent in and started the wash cycle when soapy water suddenly starts seeping out of the crevices. Your instinct in this situation is probably to stop and turn off the washing machine to investigate the problem. Likewise, cell cycle arrest occurs when the cell notices that something is wrong, and all duplication processes stop to identify the problem. If you notice a fraying, broken wire poking out from behind the wall, you will probably choose to call an electrician instead of risking a do-it-yourself fix. In the cell cycle, p53 recognizes DNA damage and activates DNA damage response pathways to initiate DNA repair. Sometimes, your machine might be too broken to fix, and you must resort to removing it from your house and heading out to buy a new one. In a cell, the analogous process is apoptosis, where a severely damaged cell is marked for destruction and effectively killed before it can do further damage or proliferate.
To investigate the relationship of p53 and Peto’s Paradox, genome-wide studies were conducted, synthesizing data from 61 animals with a range of sizes [6]. These animals included large species such as the Asian elephant and woolly mammoth. Researchers found that as species evolved to be bigger, they acquired more copies of TP53, the gene that encodes the p53 protein. While humans only have one copy of the TP53 gene, the elephant genome contains 20 copies of TP53, resulting in greater production of p53 protein. This increase may be responsible for protecting these large organisms from developing cancer [6,8]. The role of p53 has evolutionary implications because when p53 evolved as a way to regulate the cell cycle, it fortified the system in place to ensure DNA was replicated correctly and cells with mistakes were killed before the issue could spread.
Although many explanations have been proposed to account for Peto’s Paradox, more research is necessary to elucidate the precise molecular basis of the paradox. Mouse models currently dominate studies in cancer research, but they are small organisms with short life spans. Although these characteristics are useful when researchers want to conduct a study over an organism’s lifetime, they also mean that the mouse model is not the ideal model for studying cancer suppression. Expanding the study of cancer to a more diverse variety of organisms would allow for a more complete understanding of the underlying mechanisms.
Understanding Peto’s Paradox is not only important for conservation scientists and wildlife zoologists; it also has implications in human medicine. Peto’s Paradox provides an interesting opportunity for potential research that may provide insight into cancer treatment. Determining the mechanism behind cancer resistance in large animals could reveal powerful techniques to develop novel treatments for human cancers by targeting telomere length, p53 expression, or hypertumors.
References
[1] Cancer Statistics. National Cancer Institute. https://www.cancer.gov/about-cancer/understanding/statistics. Accessed December 20, 2020.
[2] Griffiths AJF, Miller JH, Suzuki DT, et al. An Introduction to Genetic Analysis. 7th edition. New York: W. H. Freeman; 2000. Mutation and cancer. Available from: https://www.ncbi.nlm.nih.gov/books/NBK21809/
[3] Caulin AF, Maley CC. Peto’s Paradox: evolution’s prescription for cancer prevention. Trends in Ecology & Evolution. 2011;26(4):175-182. doi:10.1016/j.tree.2011.01.002
[4] Nagy JD, Victor EM, Cropper JH. Why don’t all whales have cancer? A novel hypothesis resolving Peto’s paradox. Integrative and Comparative Biology. 2007;47(2):317-328. doi:10.1093/icb/icm062
[5] Are Telomeres the Key to Aging and Cancer. Learn.Genetics Genetic Science Learning Center. https://learn.genetics.utah.edu/content/basics/telomeres/. Accessed November 15, 2020.
[6] Sulak M, Fong L, Mika K, et al. TP53 copy number expansion is associated with the evolution of increased body size and an enhanced DNA damage response in elephants. eLife. 2016;5. doi:10.7554/elife.11994
[7] Olivier M, Hollstein M, Hainaut P. TP53 mutations in human cancers: origins, consequences, and clinical use. Cold Spring Harb Perspect Biol. 2010;2(1):a001008. doi:10.1101/cshperspect.a001008
[8] Abegglen LM, Caulin AF, Chan A, et al. Potential Mechanisms for Cancer Resistance in Elephants and Comparative Cellular Response to DNA Damage in Humans. JAMA. 2015;314(17):1850-1860. doi:10.1001/jama.2015.13134
by Jacky Dahlquist | Sep 15, 2020 | Genetic or Environmental?

Main Takeaways:
- Sedentary behavior (sitting or lying down for long periods of time) is harmful to your health, increasing your risk for cardiovascular disease, cancer, type 2 diabetes, and other health issues.
- While the tendency to engage in sedentary behavior appears to be somewhat heritable, it is largely influenced by your environment. This means even if you have a genetic tendency toward sedentary behavior, you aren’t destined to engage in high levels of sedentary behavior; everyone can make small changes to their routine and environment to lessen time spent sitting.
- More inclusive research in this area is needed, as well as more standardized definitions of sedentary behaviors and measures
How much time do you spend sitting each day? You have probably heard recommendations from health organizations about how much exercise you should get, how many steps you should take, or how much time you should spend looking at digital screens. However, not much is said about your sedentary behavior, or how much time you spend sitting or lying down. Sedentary behavior, which requires very little energy expenditure, is a distinct behavior from physical activity [1] . Physical activity is usually thought of as exercise; for example, you would be meeting physical activity recommendations if you ran for 30 minutes each day. Yet, even if you meet this recommendation, there is still ample opportunity to engage in sedentary behavior. Let’s say you spend an hour total commuting to and from work, sit at your office job for 8 hours, work out at the gym for 30 minutes after work, then come home and watch TV for the rest of the night until bedtime. With that routine, you would be considered physically active while still spending the majority of your waking hours sitting. How does all of this inactivity affect your health?
Sedentary behavior (SB) has repeatedly been linked to negative health impacts. A study by P. T. Katzmarzyk et al. [2] found that greater sitting time is associated with higher all-cause mortality as well as cardiovascular disease. These associations remained even in individuals that were considered physically active. In addition, a meta-analysis found positive associations between SB and cancer, type 2 diabetes, cardiovascular disease, and all-cause mortality [3]. SB has also been linked to shorter telomeres [4], organ damage, neck, shoulder, and back pain, muscle degeneration, poor circulation, and damage to discs in the spine [5].
So who is most likely to be affected by SB, and therefore more likely to suffer from these health impacts? Can you be genetically predisposed to sit more? Over the past 10 years, 8 studies have explored the heritability of SB using heritability estimates. These estimates help us to gauge a trait’s heritability; they suggest the percentage of phenotypic variability that is influenced by genetics within a population. The higher the estimate, the more influence genetics is thought to have on the trait. Heritability estimates for SB range from 7% to 56%, with an average estimate of 26% [8-15]. Twin studies were the most common form of study performed, finding heritability estimates ranging from 26%-56% [8, 13, 14, 15]. Family studies found heritability estimates ranging from 7%-41% [9, 11, 12], and the only genome-wide association study (GWAS) reported estimates of 15% (men) and 18% (women) [10].
This GWAS also discovered four loci associated with SB, near genes MEF2C, EFNA5, LOC105377146, and CALN1 [10]. These genes are related to muscle maintenance, nervous system development, and calcium binding [16-19]. Other possible candidate genes mentioned by these studies but not evaluated directly include DRD1, NHLH2, and MC4R [8]. DRD1 is related to dopamine responses and is thought to be involved with some behavioral responses, which could affect reward systems involved with activity [20]. The effect of NHLH2 on SB is less clear but seems to be related to the process of transcription [21], while MC4R produces a melanocortin hormone and is known to be a cause of monogenic obesity [22]. Each of these genes can be plausibly connected to SB; your muscles, nervous system, reward pathways, and more could all be involved in the regulation of this behavior.
Does this mean you are doomed to a sedentary lifestyle and the resulting negative health consequences if you possess variants in these genes? Luckily, that does not appear to be the case. Each of these studies promoted the idea that environmental factors have a larger influence on SB than genetics alone. This means even if you had a genetic tendency toward sedentary behavior, you wouldn’t be destined to engage in high levels of sedentary behavior; everyone can make small changes to their routine and environment to lessen time spent sitting and diminish SB’s harmful effects of the body [6]. I am currently working with Dori Rosenberg’s research team at the Kaiser Permanente Washington Health Research Institute, supporting an intervention that helps older adults make small changes in their daily lives with the goal of reducing their sitting time. By making participants more aware of how much they sit in addition to providing tools and coaching to help them sit less, this study hopes to see a decrease in SB among participants receiving the intervention. Suggestions for sitting less from the Office of Disease Prevention and Health Promotion include walking or riding a bike to get to work instead of driving, taking regular standing breaks (every 20-30 minutes) from sitting throughout the workday or while watching television, and pacing while talking on the phone instead of sitting[7] . So while studies did identify SB as a heritable trait and there are some candidate genes thought to be associated with SB [8,10], this behavior is largely affected by your environment and daily decisions. You have control over your SB regardless of your genetics.
I would be remiss, however, without describing some of the issues within existing genetic SB research. It is well known that people of non-European ancestries are continually excluded from genetic research, and a large portion of this research focuses on samples drawn from populations of European descent [23]. Six out of the eight studies I reviewed used samples of predominantly European descent [8, 9, 10, 13, 14, 15] , while the two remaining studies focused on Brazilian [11] and Portuguese [12] populations. None of the studies included representative samples of people with African, Asian, or Indigenous descent. It is important to include populations of diverse ancestries in order to increase the chances of discovering variants associated with a trait of interest, better understand the frequencies of a variant of interest in different populations, have more accurate representation of the diversity in humans [11] , and to make research more inclusive.
A second recurring limitation in the literature was the measurement of SB. The studies reviewed provided evidence that different results are seen when data are procured by self-report rather than an objective measure such as a wearable activity monitor. Downfalls of self-report are well documented [24], as this method can result in social desirability bias, recency bias, and a simple inability to accurately remember or estimate their SB. It is possible that studies using objective measurement methods may find higher heritability estimates on average — studies that used objective measurement found an average heritability estimate of 30% compared to 25% in studies that used self-report, though this comparison is based on just a few studies. For these reasons, future research should prioritize objective measurement for more accurate results. In addition, not all studies evaluated SB in the same way even within the categories of self-report and objective measurement. The lack of clear definitions and standard measures complicate comparison between studies, weakening what conclusions can be gleaned from the literature.
Despite these limitations, current evidence suggests that SB is heritable. However, it is also highly modifiable. Even if linkages between increased SB and certain gene variants were identified, knowing your genotype is unlikely to help you reduce your sitting time. This is good news — you can start making changes today to work toward sitting less, improving your overall health, and reducing your risk of chronic diseases. What are you waiting for?
References
- Sedentary Behavior Research Network. (2019, September 5). SBRN Terminology Consensus Project. Retrieved from https://www.sedentarybehaviour.org/sbrn-terminology-consensus-project/
- Katzmarzyk, P. T., Church, T. S., Craig, C. L., & Bouchard, C. (2009). Sitting Time and Mortality from All Causes, Cardiovascular Disease, and Cancer. Medicine & Science in Sports & Exercise, 41(5), 998–1005. https://doi.org/10.1249/mss.0b013e3181930355
- Biswas, A., Oh, P. I., Faulkner, G. E., Bajaj, R. R., Silver, M. A., Mitchell, M. S., & Alter, D. A. (2015). Sedentary Time and Its Association With Risk for Disease Incidence, Mortality, and Hospitalization in Adults. Annals of Internal Medicine, 162(2), 123. https://doi.org/10.7326/m14-1651
- Sjögren, P., Fisher, R., Kallings, L., Svenson, U., Roos, G., & Hellénius, M.-L. (2014). Stand up for health—avoiding sedentary behaviour might lengthen your telomeres: secondary outcomes from a physical activity RCT in older people. British Journal of Sports Medicine, 48(19), 1407–1409. https://doi.org/10.1136/bjsports-2013-093342
- Berkowitz, B., & Clark, P. (2014, January 20). The Health Hazards of Sitting. Retrieved from https://www.washingtonpost.com/gdpr-consent/?next_url=https%3a%2f%2fwww.washingtonpost.com%2fapps%2fg%2fpage%2fnational%2fthe-health-hazards-of-sitting%2f750%2f%3fitid%3dlk_inline_manual_8&itid=lk_inline_manual_8
- Barwais, F. A., Cuddihy, T. F., & Tomson, L. (2013). Physical activity, sedentary behavior and total wellness changes among sedentary adults: a 4-week randomized controlled trial. Health and Quality of Life Outcomes, 11(1), 183. https://doi.org/10.1186/1477-7525-11-183
- National Center on Health, Physical Activity and Disability. (2013, January 30). Decreasing Sedentary Behavior and Physical Inactivity by Moving More and Sitting Less. Retrieved from https://health.gov/news-archive/blog/2013/01/decreasing-sedentary-behavior-and-physical-inactivity-by-moving-more-and-sitting-less/
- den Hoed, M., Brage, S., Zhao, J. H., Westgate, K., Nessa, A., Ekelund, U., … Loos, R. J. F. (2013). Heritability of objectively assessed daily physical activity and sedentary behavior. The American Journal of Clinical Nutrition, 98(5), 1317–1325. https://doi.org/10.3945/ajcn.113.069849
- Diego, V. P., de Chaves, R. N., Blangero, J., de Souza, M. C., Santos, D., Gomes, T. N., … Maia, J. A. R. (2015). Sex-specific genetic effects in physical activity: results from a quantitative genetic analysis. BMC Medical Genetics, 16(1), 1. https://doi.org/10.1186/s12881-015-0207-9
- Doherty, A., Smith-Byrne, K., Ferreira, T., Holmes, M. V., Holmes, C., Pulit, S. L., & Lindgren, C. M. (2018). GWAS identifies 14 loci for device-measured physical activity and sleep duration. Nature Communications, 9(1), 1. https://doi.org/10.1038/s41467-018-07743-4
- Leite, J. M. R. S., Soler, J. M. P., Horimoto, A. R. V. R., Alvim, R. O., & Pereira, A. C. (2019). Heritability and Sex-Specific Genetic Effects of Self-Reported Physical Activity in a Brazilian Highly Admixed Population. Human Heredity, 84(3), 151–158. https://doi.org/10.1159/000506007
- Santos, D. M. V., Katzmarzyk, P. T., Diego, V. P., Blangero, J., Souza, M. C., Freitas, D. L., … Maia, J. A. R. (2014). Genotype by Sex and Genotype by Age Interactions with Sedentary Behavior: The Portuguese Healthy Family Study. PLoS ONE, 9(10), e110025. https://doi.org/10.1371/journal.pone.0110025
- Schutte, N. M., Huppertz, C., Doornweerd, S., Bartels, M., Geus, E. J. C., & Ploeg, H. P. (2020). Heritability of objectively assessed and self‐reported sedentary behavior. Scandinavian Journal of Medicine & Science in Sports, 30(7), 1237–1247. https://doi.org/10.1111/sms.13658
- van der Aa, N., Bartels, M., te Velde, S. J., Boomsma, D. I., de Geus, E. J. C., & Brug, J. (2012). Genetic and Environmental Influences on Individual Differences in Sedentary Behavior During Adolescence. Archives of Pediatrics & Adolescent Medicine, 166(6), 509. https://doi.org/10.1001/archpediatrics.2011.1658
- Waller, K., Vähä-Ypyä, H., Lindgren, N., Kaprio, J., Sievänen, H., & Kujala, U. M. (2018). Self-reported Fitness and Objectively Measured Physical Activity Profile Among Older Adults: A Twin Study. The Journals of Gerontology: Series A, 74(12), 1965–1972. https://doi.org/10.1093/gerona/gly263
- (2020a, June 1). LOC105377146 uncharacterized LOC105377146 [Homo sapiens (human)] Retrieved from https://www.ncbi.nlm.nih.gov/gene/?term=loc105377146
- NCBI. (2020c, June 7). CALN1 calneuron 1 [Homo sapiens (human)]. Retrieved from https://www.ncbi.nlm.nih.gov/gene/83698
- NCBI.(2020d, June 7). EFNA5 ephrin A5 [Homo sapiens (human)]. Retrieved from https://www.ncbi.nlm.nih.gov/gene/1946
- NCBI. (2020g, June 28). MEF2C myocyte enhancer factor 2C [Homo sapiens (human)]. Retrieved from https://www.ncbi.nlm.nih.gov/gene/4208
- NCBI.(2020b, June 4). DRD1 dopamine receptor D1 [Homo sapiens (human)]. Retrieved from https://www.ncbi.nlm.nih.gov/gene/1812
- NCBI. (2020e, June 7). NHLH2 nescient helix-loop-helix 2 [Homo sapiens (human)]. Retrieved from https://www.ncbi.nlm.nih.gov/gene/4808
- NCBI. (2020f, June 27). MC4R melanocortin 4 receptor [Homo sapiens (human)]. Retrieved from https://www.ncbi.nlm.nih.gov/gene/4160
- Genetics for all. (2019). Nature Genetics, 51(4), 579. https://doi.org/10.1038/s41588-019-0394-y
- Rosenman, R., Tennekoon, V., & Hill, L. G. (2011). Measuring bias in self-reported data. International Journal of Behavioural and Healthcare Research, 2(4), 320. https://doi.org/10.1504/ijbhr.2011.043414
by Diane Xue | Jun 1, 2020 | Disease Genetics, Genetic Misconceptions
Main Takeaways:
- Late-onset Alzheimer’s Disease (LOAD) is influenced by many genetic and environmental risk factors. There is no gene that guarantees onset or immunity from (LOAD).
- At-home genetic testing companies that offer an “Alzheimer’s test” are only testing one variant from one gene (APOE). The presence or absence of this APOEε4 variant is not a diagnosis.
- Thus far, over two dozen risk loci for LOAD have been discovered, yet a genetic test is not sufficient to detect one’s true risk of developing disease.
When I was seven years old, my parents took me back to their hometown of Shanghai to visit my grandparents. It was a long trip we had taken before, and while the 18-hour journey was tiring, I was looking forward to seeing family, eating street food, and finding cats in the park. This trip was different, however. My mom explained to me that my grandpa may not remember us. He has Alzheimer’s, she said. Although I didn’t know what that meant, it made sense to me that my grandpa may not remember me. After all, I had changed a lot between three and seven. When we finally arrived at my grandparents’ home, I realized that it wasn’t that my grandpa was forgetful; there was a blankness. He couldn’t leave his bed.
My grandpa was suffering from a neurodegenerative disease – meaning it affects the brain and becomes worse over time. Alzheimer’s disease is the most common cause of dementia, affecting an estimated 5.8 million people in the United States and 1 out of every 10 people over age 65 [1]. The disease is devastating for those affected, their caregivers, and their loved ones. When 23andMe and other at-home (direct-to-consumer) genetic testing companies began testing for Alzheimer’s disease risk, I understood the impulse to spit in a tube and find out one’s genetic risk for the disease [2]. That impulse is driven by the feeling of fear that immediately follows annoyance when my mom turns the car back around two minutes after leaving the house because she can’t remember if she left the stove on. Of course, I want to know my risk for disease and everything I can do to prevent it. The fact is, that’s just not possible yet.
That impulse to order the genetic test is also based on the misconception that all of the genetic causes of Alzheimer’s disease are known. Last year Cosmopolitan published an op-ed titled “I tested positive for the Alzheimer’s Gene at 26 years old,” where the author shared her emotional experiences after receiving her genetic testing results and the lifestyle changes she made to help prevent the disease [3]. While I don’t believe genetic testing companies are trying to be scientifically deceiving, the op-ed highlights the consequences and unwarranted anxiety that can occur when the science and genetics of Alzheimer’s disease are not clearly presented. In this post, I will share the facts about Alzheimer’s Disease genetics. (For the rest of this post, Alzheimer’s Disease will be referred to as AD).
There are two classifications of AD: early-onset AD and late-onset AD. Early-onset AD is rare. It accounts for about 1% of all AD cases [4]. Unlike most AD cases, the genetic causes of early-onset AD are known; it is caused by mutations in the genes APP, PSEN1, or PSEN2. Early-onset AD is transmitted in a dominant Mendelian pattern. For every gene, you receive one copy (allele) from your father and one from your mother. A dominant Mendelian pattern means that if you receive just one copy with a mutation, you will develop the disease. Once again, I want to reiterate that this type of AD is very rare. If you have a family history of early-onset AD and are not in contact with a medical provider or research group, you can find more information and resources here [5].
The more common version of AD, the type that my grandpa had and the version most direct-to-consumer genetic testing companies offer a test for, is late-onset AD. Unlike the early-onset version, late-onset AD is a complex, genetically heterogeneous trait, meaning it is influenced by both environmental and genetic factors. Furthermore, different genetic variants cause the disease in different people. In other words, there is no “Alzheimer’s gene.” There are many variants in many genes that add to one’s genetic risk, and many risk variants have not been discovered yet.
So why the hype? What is the scientific basis for the direct-to-consumer late-onset AD tests that have resulted in online forums, Facebook support groups, and op-eds in popular magazines?
The direct-to-consumer tests calculate genetic risk based on the variation in a single gene: APOE. Everyone has the APOE gene on chromosome 19; what matters for AD risk is which variants of the gene you have [6]. There are three variants (six possible combinations): ε2, ε3, and ε4. APOE ε2 has been found to have protective effects, while APOE ε4 is the variant associated with an increased risk of late-onset AD. Studies have found that on average, having one copy of ε4 increases the risk for late-onset AD by 3-fold, and having two APOE ε4 alleles increases risk by 12-fold [4].
I am not trying to dismiss concerns over this increased risk; three times higher and twelve times higher risk are significant. APOE ε4 is the strongest genetic risk factor for late-onset AD that we know of. However, it is important to keep the following in mind:
(1) Inheriting two copies of APOE ε4 is rare, affecting only 2-3% of the population (see image below) [4].
(2) Late-onset AD is influenced by genetic risk factors AND environmental factors, with one study showing that 47% of the variance of AD is due to non-genetic factors [7].
(3) Having two copies of APOE ε4 is not an Alzheimer’s disease diagnosis, nor is the absence of APOE ε4 a guarantee that you will not get AD. Environmental factors aside, there are many other genetic variants that confer risk for late-onset AD (see image below).
(4) Finally, the overwhelming majority (over 90%) of research on the genetic risks of Alzheimer’s have been conducted in samples of people of non-Hispanic European descent [8]. The few studies of APOE risk on AD in diverse populations have shown that the risk of AD caused by the APOE ε4 allele varies depending on ancestry. In 2019, a study of late-onset AD in Caribbean Hispanics found that individuals with African-derived ε4 allele at the APOE gene had 39% lower odds of having AD than those with a European-ancestry-derived copy of ε4 [9].
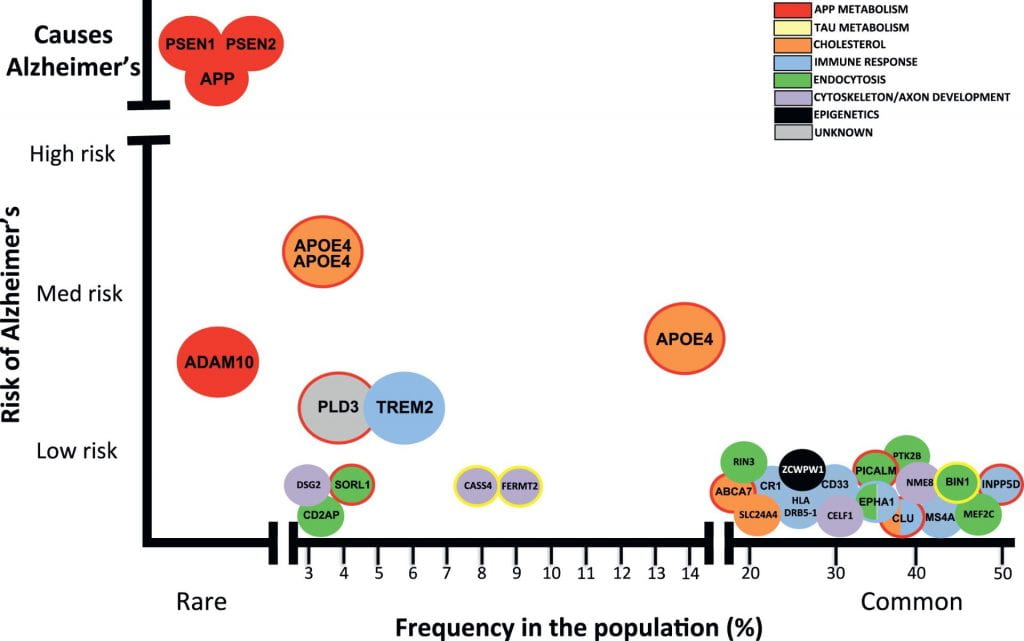
Image from Celeste M. Karch, and Alison M. Goate, “Alzheimer’s Disease Risk Genes and Mechanisms of Disease Pathogenesis.” Alzheimer’s risk-variants are plotted with frequency in the population on the X-axis and the risk conferred by each variant on the Y-axis. Mutations in PSEN1, PSEN2, and APP cause early-onset Alzheimer’s disease. Dozens of genetic mutations have been confirmed to increase risk of late-onset Alzheimer’s disease, with APOE ε4 being the strongest known disease-variant.
The bottom line is that these direct-to-consumer genetic tests of late-onset AD can tell you one piece about your risk for disease, but it can’t factor in how APOE interacts with other genes or with your environment; the test is based primarily on research of those with European ancestry; and it cannot tell you whether or not you will have Alzheimer’s disease. I look forward to the day when I can edit this post to say that an accurate assessment for late-onset AD genetic risk exists, but we’re not quite there yet. (note: if you are thinking about ordering an direct-to-consumer genetic test for AD, the Alzheimer’s Association strongly recommends you consult with a genetic counselor before the test and after receiving your results).
That trip in 2004 was the last time I saw my grandpa. At the time, I didn’t know what was happening, but I could feel the hopelessness in the air. It hits kids hard when the adults in the room are saying they don’t know anything more, that nothing can be done. I understand that it can seem discouraging that there is so much we still don’t know, that there is still no cure. But I am not hopeless anymore. In 2004, studies of the human genome were in its infancy, with The Human Genome Project having been completed only months before. At the time, there had been no genome-wide studies of Alzheimer’s disease. In the past 15 years alone, 40 genomic regions that are associated with late-onset AD have been found [10]. Every year, we learn more about new risk variants and biological pathways that can be targeted for pharmaceutical therapies. Significant efforts are being put toward researching the genetics of Alzheimer’s, worldwide, with the hope that one day we will understand the entire genetic architecture of the disease. I am optimistic for what the next 15 years will bring.
References:
[1] 2020 Alzheimer’s Disease Facts and Figures. Alzheimer’s Association. Retrieved from https://www.alz.org/media/Documents/alzheimers-facts-and-figures.pdf
[2] Late-onset Alzheimer’s Disease. 23andMe. Retrieved from https://www.23andme.com/topics/health-predispositions/late-onset-alzheimers/
[3] Brown, S. “I Tested Positive for the Alzheimer’s Gene at 26 years old.” Cosmopolitan Sep. 19, 2019. Retrieved from https://www.cosmopolitan.com/health-fitness/a29107622/alzheimers-gene/
[4] Karch, Celeste M. and Alison M. Goate. 2015. Alzheimer’s Disease Risk Genes and Mechanisms of Disease Pathogenesis. Vol. 77. doi:https://doi.org/10.1016/j.biopsych.2014.05.006. http://www.sciencedirect.com/science/article/pii/S0006322314003394.
[5] Younger/Early-Onset Alzheimer’s. Alzheimer’s Association. Retrieved from https://www.alz.org/alzheimers-dementia/what-is-alzheimers/younger-early-onset
[6] Roses, Allen D. and Ann M. Saunders. 1994. APOE is a Major Susceptibility Gene for Alzheimer’s Disease. Vol. 5. doi:https://doi.org/10.1016/0958-1669(94)90091-4. http://www.sciencedirect.com/science/article/pii/0958166994900914.
[7] Ridge, PG, Hoyt, KB, Boehme, K et al. 2016. Assessment of the genetic variance of late-onset Alzheimer’s disease. Neurobiol Aging. Vol. 41 doi.org/10.1016/j.neurobiolaging.2016.02.024.
[8] Popejoy, AB, and Fullerton, SM. 2016. Genomics is failing on diversity. Nature, 538(7624), 161–164. https://doi.org/10.1038/538161a
[9] Blue EE, Horimoto ARVR, Mukherjee S, Wijsman EM, Thornton TA. 2019. Local ancestry at APOE modifies Alzheimer’s disease risk in Caribbean Hispanics. Alzheimer’s & Dementia: the Journal of the Alzheimer’s Association. Vol. 12. doi.org/10.1016/j.jalz.2019.07.016
[10] Andrews, Shea J., Brian Fulton-Howard, and Alison Goate. 2020. Interpretation of Risk Loci from Genome-Wide Association Studies of Alzheimer’s Disease. Vol. 19. doi:https://doi.org/10.1016/S1474-4422(19)30435-1. http://www.sciencedirect.com/science/article/pii/S1474442219304351.
by Hanley Kingston | Apr 28, 2020 | Evolution
Main Takeaways:
- Neurological diseases and disorders that strike during young age present some fascinating questions. 1) Is there a link between a genetic predisposition to these conditions and a propensity for genius or creative talent? 2) How have these often debilitating conditions remained so common, when many evolutionary models suggest the genetic risk factors for these diseases would have been eliminated through evolution?
- New research shows that many early-acting hereditary neurological diseases are caused by similar types of genetic mutations. The occurrence of these mutations is common, but the exact location of each mutation can be unique to a small number of families. It is the commonality with which these mutations occur that explains the commonality of neurological diseases and disorders like schizophrenia, bipolar disorder, and autism, largely independently of the “genius connection.”
- Humans may be unique in their propensity to develop such mutations.
The most basic principles of evolution dictate that serious genetic diseases that affect the young are rare because their sufferers are often less likely to have children and, therefore, less likely to pass along the disease-causing mutation(s). But common neurological diseases and disorders like schizophrenia, bipolar disorder, and autism long confused evolutionary geneticists by defying this principle. For instance, although most people with schizophrenia do not have children, the disease remains both highly heritable and common: striking about one in every hundred people.[1]
These conditions are fascinating, not only for their hard-to-explain abundance, but because of another well-known paradox – an apparent excess of savantism and creative genius among people affected by these neurological conditions, as well as among their family members. But while early hypotheses suggested that such cases might offer unique insight into the genetics and evolution of human cognition, the findings were often soured by preconceived notions about class and intelligence, from a 1970 study that observed that “close [male] relatives of psychotic individuals have a significantly increased probability of being considered persons of eminence” to more recent studies that crudely use occupation and college major as proxies for creative talent.[2]
Captivated by the possibility of a connection between genius and neurological disease, however, early research proposed that a long-understood evolutionary phenomenon called balancing selection, might explain the commonality of schizophrenia and autism. In balancing selection, inherited parts of the DNA that cause disease in some people are able to last throughout many generations by benefiting others. Sickle Cell Disease is a well-known example. People who have two copies of a mutation in the hemoglobin-beta gene (one from each parent) suffer from the life-threatening disease. People who carry just one copy, on the other hand, have few of the associated negative health effects but do have enough of the sickle-shaped blood cells to make their blood resistant to infection by malaria-causing parasites. By affording resistance to a different disease, the mutation for sickle cell increases its own odds of making it into the next generation and bucks the forces of evolution that would otherwise largely eliminate it. The authors of the 1970 study on schizophrenia and eminence postulated a very similar mechanism – the existence of at least one genetic mutation that could cause schizophrenia in people who inherited it from both parents but which increased creative talent in people who inherited only the one copy.[2]
As genetic causes of many diseases were solved through advanced genetic techniques, however, it became clear that most neurological diseases were not quite like Sickle Cell Disease in that not everyone with the disease had a mutation in the same gene. In fact, very few common diseases, neurological or otherwise, can be explained by the same mutation in each person.
Most common diseases are caused by a combination of many common variations between different people’s DNA (termed genetic variants).[3] Everyone carries around some pieces of DNA that increase their risk of diseases and others that decrease their risk. In certain families and, therefore, certain individuals, the risk-increasing pieces for a given disease are especially common, and they are more likely to develop the full-blown disease. This is the genetic mechanism that best explains asthma and cardiovascular disease and has proven roughly accurate to explain late-onset neurological conditions like Parkinson’s and Alzheimer’s. Because Parkinson’s and Alzheimer’s usually occur in late adulthood, however, they have little effect on the likelihood of having children and their commonality is not paradoxical, as it is for diseases like schizophrenia, where we would not expect harmful variants to persist throughout generations. Among other popular explanations, we return to balancing selection, which can also apply to diseases caused by multitudes of common variants if the common variants are beneficial to the people who dodged the full-blown disease. The variants, scattered throughout the genome, are hypothesized to act like vitamins or drugs: they’re helpful, to a point – but detrimental in too high a dose.
Balancing selection explains many aspects of human physiology, but as an explanation of the persistence of common neurological disorders, the theory has recently suffered several major blows. Most seriously, there is little indication that genius of any variety yields any advantage from an evolutionary standpoint. Selection depends on the number and health of your children and is largely indifferent to Who Is Who lists.[4] This does not mean that the “mad genius” connection is not real or that it has nothing to tell us about the evolution of the human brain; recent research suggests that the link between schizophrenia and creativity, if present, has been overblown, but there’s likely some link between bipolar disorder an IQ and savantism, while rare, is overrepresented in autistic individuals.[4,5,6,7,8] While modern research remains mixed on whether there is a connection between neurological disorders and specific skillsets, it is almost definitely not the driving force behind the prevalence of these diseases.
Recent research finally explains common neurological conditions in a way that is consistent with long-established principles of evolution. Like sickle cell disease, these conditions are often caused by a single mutation in each person, but unlike sickle cell disease, it is not the same mutation in each person.[9] Like a boat flooding as fast as it is bailed out, every generation, new mutations pop up randomly in different spots in different unlucky people.
The impact of common rare mutations is not unique to neurological disease. Cancer-stricken families are often uniquely unlucky in that the mutations they share are very uncommon outside of their family, although hereditary cancers as a whole are sadly abundant. Medical practice is just starting to recognize the importance of considering these distinct mutations in classifying and treating cancers. Similarly, Schizophrenia disease-causing mutations are scattered throughout the genome, yet they cluster by the types of biological pathways they fall into.[9] Similarly, researchers have been able to subclassify cases of autism – a hugely broad umbrella diagnosis – by their rare mutations, finding subsets of ten or twenty patients from around the world who share the same mutations and who show pronounced similarities in appearance, mannerisms, and health.[10] These studies are also resolving a third paradox of neurological disease – a tendency for families to experience high levels of more than one prognostically distinct neurological disease. It turns out that the genes in which the disease-causing mutations occur are often the same genes that give rise to mutations causing other neurological conditions like depression, bipolar disorder, autism, epilepsy, and schizophrenia.[ 11,12]
On its face, this conclusion seems less than extraordinary: neurological disorders are common because our brains are complex and typical function depends on a large percentage of our genes working properly; however, this simple finding has much to teach us about the evolution of the human brain.[9] How did humans get to be so sensitive to these mutations? Our genome may be uniquely fragile. While humans are not special in our number of genes – we have fewer than some fleas – we are unique in the number of segments in our DNA that have nearly exact copies in separate parts of our genome. Certain areas of the genome are hotspots for co-opting these duplicated segments and occasionally merge them into novel genes. Creating new “patchwork” genes in this way may have given us a cognitive edge over other primates; however, the consequence of having parts of our genome that almost exactly match other parts is that sometimes the replication machinery in the genome gets confused between the two regions and causes errors, like failing to copy part of the DNA or copying a part too many times. These errors can result in both common and rare diseases that are often neurological in nature.[13,14] The errors that occur between duplicated regions look distinct from the types of errors that cause diseases like sickle-cell or the genetic variants that increase the risk of Alzheimer’s disease. This new research suggests neurological diseases may be a price we pay for evolving the most advanced brains in the animal kingdom.
References:
- Keller, M. C. (2008). The evolutionary persistence of genes that increase mental disorders risk. Current Directions in Psychological Science, 17(6), 395-399.
- KARLSSON, J. L. (1970). Genetic association of giftedness and creativity with schizophrenia. Hereditas, 66(2), 177-181.
- https://en.wikipedia.org/wiki/Common_disease-common_variant
- Greenwood, T. A. (2016). Positive traits in the bipolar spectrum: the space between madness and genius. Molecular neuropsychiatry, 2(4), 198-212.
- Pearlson, G. D., & Folley, B. S. (2008). Schizophrenia, psychiatric genetics, and Darwinian psychiatry: an evolutionary framework. Schizophrenia bulletin, 34(4), 722-733.
- Folley, B. S., & Park, S. (2005). Verbal creativity and schizotypal personality in relation to prefrontal hemispheric laterality: A behavioral and near-infrared optical imaging study. Schizophrenia research, 80(2-3), 271-282.
- Keller, M. C., & Visscher, P. M. (2015). Genetic variation links creativity to psychiatric disorders. Nature neuroscience, 18(7), 928-929.
- https://www.spectrumnews.org/features/deep-dive/extraordinary-minds-the-link-between-savantism-and-autism/
- Walsh, T., McClellan, J. M., McCarthy, S. E., Addington, A. M., Pierce, S. B., Cooper, G. M., … & Stray, S. M. (2008). Rare structural variants disrupt multiple genes in neurodevelopmental pathways in schizophrenia. science, 320(5875), 539-543.
- Sebat, J., Lakshmi, B., Malhotra, D., Troge, J., Lese-Martin, C., Walsh, T., … & Leotta, A. (2007). Strong association of de novo copy number mutations with autism. Science, 316(5823), 445-449.
- https://www.spectrumnews.org/news/schizophrenia-prevalence-may-threefold-higher-people-autism/
- https://www.nimh.nih.gov/news/science-news/2018/suspect-molecules-overlap-in-autism-schizophrenia-bipolar-disorder.shtml
- Sharp, A. J., Hansen, S., Selzer, R. R., Cheng, Z., Regan, R., Hurst, J. A., … & Fitzpatrick, C. A. (2006). Discovery of previously unidentified genomic disorders from the duplication architecture of the human genome. Nature genetics, 38(9), 1038-1042.
- Mefford, H. C., & Eichler, E. E. (2009). Duplication hotspots, rare genomic disorders, and common disease. Current opinion in genetics & development, 19(3), 196-204.
Recent Comments