Research
We are broadly interested in understanding how large macromolecular complexes assemble, and how assembly contributes to intracellular organization. We primarily use cryo-EM to determine high resolution structures of protein assemblies, and complement our structural work with biochemical, biophysical, and cell biological experiments.
Structure and function of polymerizing enzymes
Intermediate metabolism is finely tuned, carefully balanced, and robustly adaptable to changes in environmental conditions. While the individual enzymes that drive most metabolic processes are well understood, only recently has the field come to appreciate the widespread role of metabolic enzyme self-assembly in metabolic organization and control. We now know that dozens of metabolic enzymes assemble into filamentous structures in vivo and in vitro, and that these filaments act as allosteric effectors to tune enzyme activity. We focus on a number of important enzymes in different metabolic pathways, with a major focus on nucleotide biosynthesis and glycolysis. Many of these are also important drug targets, and we have recently begun efforts to understand the function of drugs in the context of filament assembly, with the goal of improving therapeutic outcomes.
Collaborators: Ethan Garner (Harvard University), Jeff Peterson (Fox Chase Cancer Center), Brad Webb (West Virginia University), Peter Swain (University of Edinburgh), Nimbus Therapeutics (Cambridge, MA), Hesper Rego (Yale University)
Representative recent publications:
IMPDH1 retinal variants control filament architecture to tune allosteric regulation
Burrell AL, Nie C, Said M, Simonet JC, Fernández-Justel D, Johnson MC, Quispe J, Buey RM, Peterson JR, Kollman JM (2022) Nature Structural and Molecular BiologyCryo-EM Structures of CTP Synthase Filaments Reveal Mechanism of pH-Sensitive Assembly During Budding Yeast Starvation
Hansen JM, Horowitz A, Lynch EM, Farrell DP, Quispe J, DiMaio F, Kollman JM (2021) eLifeStructural basis for isoform-specific inhibition of human CTPS1
Lynch EM, DiMattia MA, Albanese S, van Zundert GCP, Hansen JM, Quispe JD, Kennedy MA, Verras A, Borrelli K, Toms AV, Kaila N, Kreutter KD, McElwee JJ, Kollman JM (2021) Proceedings of the National Academy of Sciences USAIndependent evolution of polymerization in the Actin ATPase clan regulates hexokinase activity.
Stoddard PR, Lynch EM, Farrell DP, Dosey AM, William TA, Kollman JM, Murray AW, Garner EC (2020) ScienceCryo-EM structures demonstrate human IMPDH2 filament assembly tunes allosteric regulation.
Johnson MC, Kollman JM (2020) eLifeCoupled structural transitions enable highly cooperative regulation of human CTPS2 filaments.
Lynch EM, Kollman JM (2020) Nature Structural and Molecular Biology
Cytoskeleton

Filament structure of AlfA, a bacterial actin that segregates plasmids. (Usluer, et al. 2018)
Prof. Kollman studied the mechanisms of microtubule nucleation during his postdoctoral work, and the lab is now interested in a diverse set of cytoskeletal filaments. Our main emphasis has been on highly divergent actins, including specialized actins from bacteria that are involved in plasmid segregation and organelle positioning. Ongoing efforts are focused on understanding the most divergent Eukaryotic actin, from Giardia lamblia, a human intestinal parasite. We also study non-traditional bacterial cytoskeletal filaments, like bactofilins,
Collaborators: Alex Paredez (University of Washington), Dyche Mullins (UC San Francisco), Arash Komeili (UC Berkeley), Zemer Gitai (Princeton University), Nina Salama (Fred Hutchinson Cancer Research Center), Harry Higgs (Dartmouth College) Frank DiMaio (University of Washington)
Representative publications:
Independent evolution of polymerization in the Actin ATPase clan regulates hexokinase activity.
Stoddard PR, Lynch EM, Farrell DP, Dosey AM, William TA, Kollman JM, Murray AW, Garner EC (2020) ScienceThe Structure and Dynamics of C. elegans Tubulin Reveals the Mechanistic Basis of Microtubule Growth.
Chaban S, Jariwala S, Hsu C-T, Redemann S, Kollman JM, Müller-Reicher T, Sept D, Bui KH, Brouhard GJ (2018) Developmental CellCryo-EM structure of the bacterial actin AlfA reveals unique assembly and ATP-binding interactions and the absence of a conserved subdomain.
Usluer G, Dimaio F, Yang S, Hansen JM, Polka JK, Mullins RD, Kollman JM (2018) PNASThe tetrameric kinesin Kif25 suppresses pre-mitotic centrosome separation to establish proper spindle orientation.
Decarreau J, Wagenbach M, Lynch E, Halpern AR, Vaughn JC, Kollman JM, Wordeman L (2017) Nature Cell BiologyStructure of the magnetosome-associated actin-like MamK filament at subnanometer resolution.
Bergeron JR, Hutto R, Ozyamak E, Hom N, Hansen J, Draper O, Byrne ME, Keyhani S, Komeili A, Kollman JM (2017) Protein Science
Bacterial Machines
We work on a number of nanomachines involved in bacterial cell biology. Major current efforts are focused on the R-body, an amazing coiled piston-like system used in interspecies cellular warfare. The coiled structure gets taken up into food vacuoles of sensitive strains of paramecium, and upon acidification in the vacuole an enormous pH-induced conformational change results in rapid extension of the R-body that ruptures the vacuole membrane and allows delivery of toxins directly into the target cell. We are using a combination of structural and biophysical approaches to understand the mechanism of R-body conformational change, and measure the forces it can generate.
Collaborators: Chip Asbury (University of Washington), Miklos Guttman (University of Washington), Julien Bergeron (King’s College, London), Antoine Loquet (University of Bordeaux), James DeYoreo (University of Washington), Sam Miller (University of Washington)
Representative recent publications
Lysogenic symbiosis in SAR11.
Morris RM, Cain KR, Hvorecny KL, Kollman JM (2020) Nature MicrobiologyThe Acinetobacter baumannii Mla system and glycerophospholipid transport to the outer membrane.
Kamischke C, Fan J, Bergeron J, Kulasekara HD, Dalebroux ZD, Burrell A, Kollman JM, Miller SI (2019) eLife
Protein Design
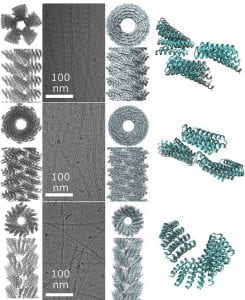
De novo designed helical filaments. Designs (left) and cryo-EM structures (right). (Chen, et al. 2018)
We collaborate with members of the Institute for Protein Design to determine structures of de novo designed self-assembling structures like filaments, arrays, pores, and motors. These designs have enormous potential for applications in medicine, energy, and nanotechnology.
Collaborators: David Baker (University of Washington), Frank DiMaio (University of Washington)
Representative recent publications:
Design of biologically active binary protein 2D materials.
Ben-Sasson AJ, Watson J, Sheffler W, Johnson MC, Bittleston A, Logeshwaran S, Decarreau J, Jiao F, Chen J, Mela I, Drabek AA, Jarrett SM, Blacklow SC, Kaminski CF, Hura GL, De Yoreo JJ, Kollman JM, Ruohola-Baker H, Delivery E, Baker D (2021) NatureComputational design of transmembrane pores.
Xu C, Lu P, Gamal TM, Pei X-Y, Johnson MC, Uyeda A, Bick MJ, Xu Q, Jiang D, Bai H, Reggiano G, Yang H, Brunette TJ, Dou J, Ma D, Lynch EM, Boyken SE, Huang PS, Stewart L, DiMaio F, Kollman JM, Luisi BF, Matsuura T, Catterall WA, Baker D (2020) NatureModular repeat protein sculpting using rigid helical junctions.
Brunette TJ, Bick MJ, Hansen JM, Kollman JM, Baker D (2020) PNASSelf-assembling 2D arrays with de novo protein building blocks.
Chen Z, Johnson MC, Chen J, Bick MJ, Boyken SE, Lin B, De Yoreo JJ, Kollman JM, Baker D, DiMaio F (2019) JACSDe novo design of self-assembling helical protein filaments.
Shen H, Fallas JA, Lynch E, Sheffler W, Parry B, Jannetty N, Decarreau J, Wagenbach M, Chen J, Wang L, Dowling Q, Oberdorfer G, De Yoreo J, Wordeman L, Jacobs-Wagner C, Kollman JM, Baker D. (2018) Science